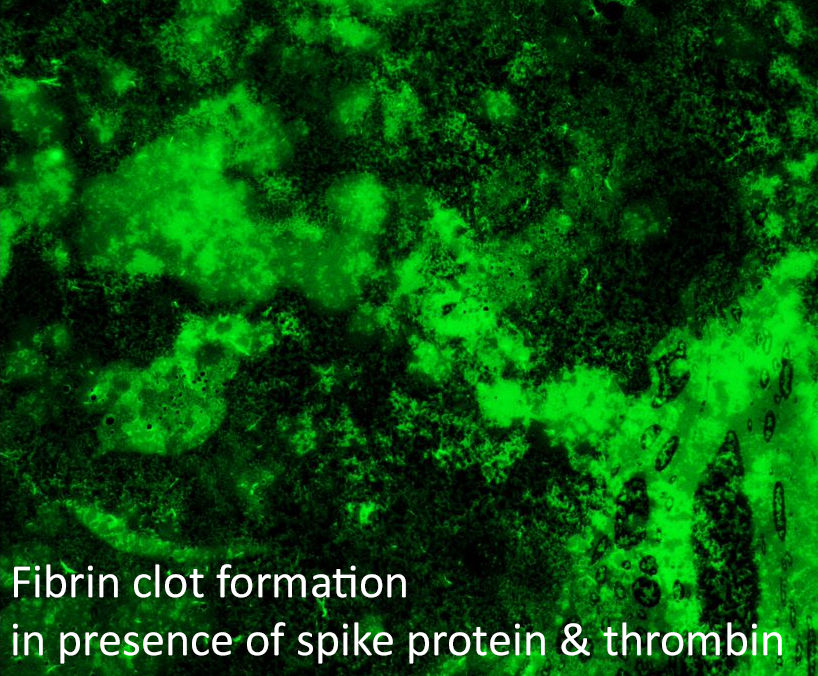
As research into the pathologies of COVID-19 advanced, we soon understood that microthrombi or microclots in the lungs of COVID-19 patients was a hallmark sign of the disease. In a recent article "SARS-CoV-2 spike protein S1 induces fibrin(ogen) resistant to fibrinolysis: Implications for microclot formation in COVID-19" from Lize M. Grobbelaar et al. [1]; researchers used Cellix's standardised Vena8 Fluoro+ biochips and microfluidic syringe pumps to model microclot formation where samples included platelet poor plasma (PPP) from COVID-19 patients and PPP from healthy patients with spike protein subunit 1 (S1) added. Spike protein is a membrane glycoprotein found on the Coronavirus. This S1 subunit of the spike protein is responsible for receptor binding, [2]. Further paper publications have shown that the spike protein can be shed by the virus and it has been found in various organs, even crossing the blood-brain barrier, [3].
Researchers set out to investigate if the spike protein interfered with blood flow, comparing naïve healthy PPP samples, with and without added spike protein, to PPP samples from COVID-19 positive patients (before treatment). Let's take a closer look at the researchers' experimental set-up, how they modelled microclot formation and what they learned in the process:
Standard Experimental set-up
Using Cellix's VenaFlux platform which includes a microfluidic syringe pump (e.g. Mirus Evo syringe pump) and Vena8 Fluoro+ biochips:
Microchannel of the Vena8 Fluoro+ biochip was flushed with distilled water at 1mL/min for 1 minute.
Microchannels were coated with thrombin: thrombin was pumped through the microchannel at 50μL/min for 90 seconds; then left to stand for 5 minutes.
The sample (control, control with spike protein or COVID-19) was pumped at a flow rate or 10μL/min for 5 minutes while a video recorded the microclot formations.
After the flow was stopped, a set of images was taken. The sample was left for another 5 minutes to enable observation of any further changes and another set of images was taken.
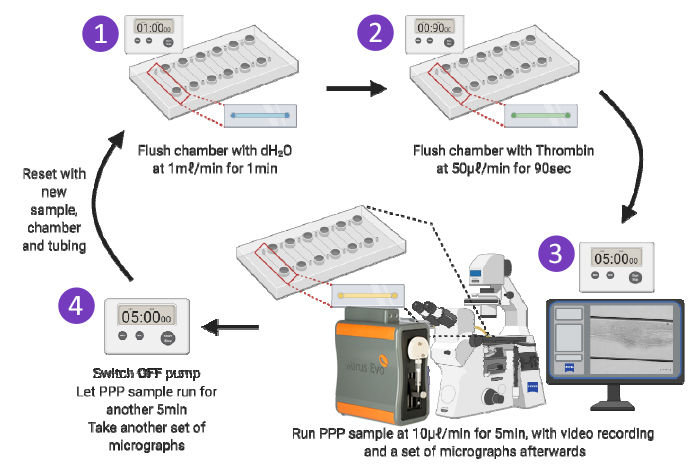
Results & Discussion

Figure 7 from the article by Grobbelaar et al. [1]; shows the microclots formed within the thrombin-coated microchannel of the Vena8 Fluoro+ biochip.
A) Healthy PPP clot with a small clot formation (arrow), with B) no clot formed in the healthy PPP sample;
C and D) examples of clots from COVID-19 PPP samples and
E and F) healthy PPP clot with spike protein added.
As illustrated in Figure 7 from this article, there was little or no clot formation in healthy PPP samples. The microclot observed in A) was positioned at the walls of the microchannel - this is more consistent with regular healthy blood flow where unactivated (bi-convex disc-shaped) platelets flow alongside the vessel wall. In contrast, the COVID-19 PPP samples in C and D) display clots that cover a significant area within the microchannel, the dimensions of which is 400μm wide and 100μm in depth. The researchers noted in their article that the formation of these clots was rapid, within 90 seconds but following their formation, they did not spread much beyond that. Researchers went on to explain that clot formation of the healthy PPP sample with added spike protein in E and F)"demonstrated a bridge between healthy PPP clots and COVID-19 clots" with " clumped clot areas, co-existing with laminar fibrous PPP clots".
If a clot breaks loose and travels through the bloodstream, it is called a thromboembolism which can lead to various life-threatening conditions. The beauty of a flow-based system is that by experimenting with different flow rates (shear rates or shear stresses), we can investigate how easily a clot may be dislodged once it has formed within the microchannel of the biochip.
In the work carried out by these researchers, they commented that clots from healthy PPP, as seen in A) and larger clots from healthy PPP with added spike protein as seen in E and F), could easily be dislodged by flushing the flow channel with water at a rate of 1mL/min. However this was not possible with the clots formed by samples from COVID-19 patients, C and D). In this latter case, even when high flow rates were applied, the clots could not be dislodged and they remained intact and in place.
Conclusions
With respect to the work related to the flow studies in this highlighted article, the researchers suggested that, in part, "the presence of spike protein in circulation may contribute to the hypercoagulation in COVID-19 positive patients and may cause severe impairment of fibrinolysis". The researchers concluded that the spike protein may have pathological effects directly - without having to be taken up by the cells. Thus, they suggest that it provides further evidence that targeting it directly, whether via vaccines or antibodies, is likely to be of therapeutic benefit.
If you'd like to learn more about Cellix's VenaFlux Solutions for similar studies, contact us now, we'd be happy to help you find the best solution for your budget.
Vena8 Fluoro+ biochips
Vena8 Fluoro+ biochips were used by the researchers in the study above. These chips are ideal for platelet adhesion and aggregation shear flow experiments, particularly at high shear rates. The chip is robust, simple, and easy to use. Click here to find out more.
[1] Lize M. Grobbelaar et al. SARS-CoV-2 spike protein S1 induces fibrin(ogen) resistant to fibrinolysis: Implications for microclot formation in COVID-19. medRxiv. 2021 Mar; doi: https://doi.org/10.1101/2021.03.05.21252960 [2] Yasunori Watanabe et al. Site-specific glycan analysis of the SARS-CoV-2 spike. Science 17 Jul 2020: Vol. 369, Issue 6501, pp. 330-333. DOI: 10.1126/science.abb9983 [3] Rhea, E.M., Logsdon, A.F., Hansen, K.M. et al. The S1 protein of SARS-CoV-2 crosses the blood–brain barrier in mice. Nat Neurosci24, 368–378 (2021). https://doi.org/10.1038/s41593-020-00771-8